Post-Quantum Cryptography
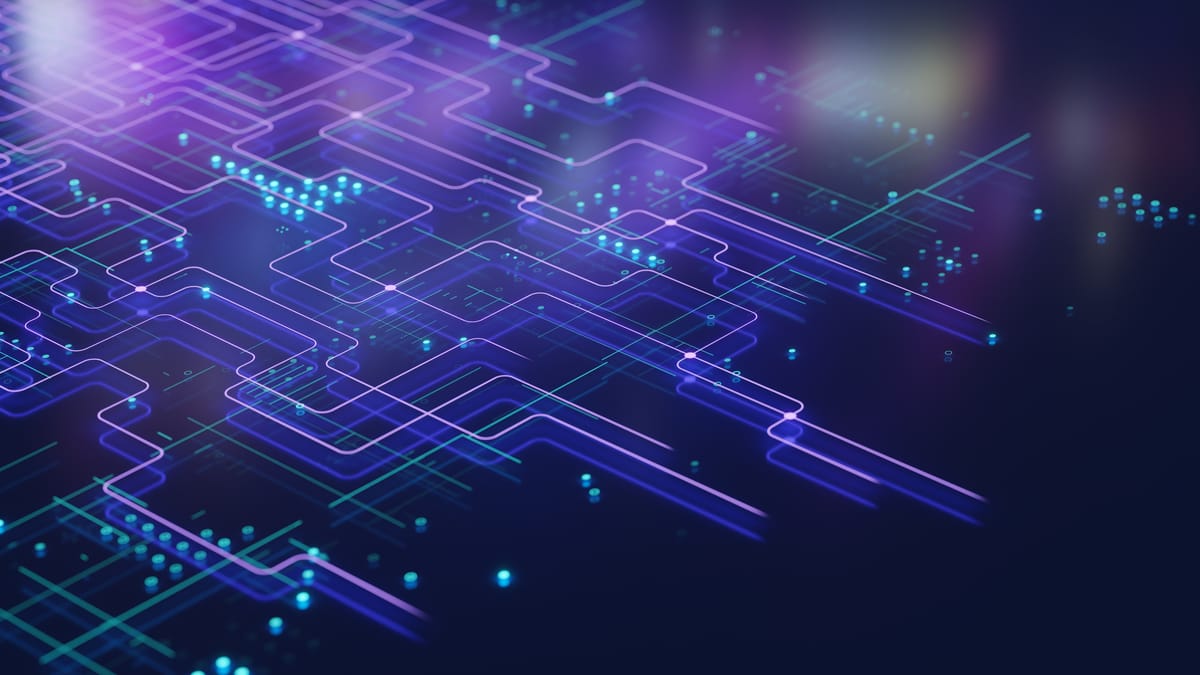
Post-Quantum Cryptography: Securing National Communications for the Quantum Era
Post-quantum cryptography (PQC) comprises cryptographic algorithms designed to resist attacks by quantum computers, which threaten to break widely used public-key schemes—such as RSA and ECC—through Shor’s algorithm. By relying on mathematical problems believed to be intractable even for large-scale quantum devices, PQC ensures the long-term confidentiality and integrity of government, military, and critical-infrastructure communications. As nations race to field quantum computing capabilities, establishing robust PQC standards and implementations has become a strategic imperative to protect tomorrow’s sensitive data and maintain technological sovereignty.
At the heart of PQC are several algorithm families, each grounded in distinct hard problems. Lattice-based schemes, like CRYSTALS-Kyber for key encapsulation and CRYSTALS-Dilithium for digital signatures, leverage the difficulty of finding short vectors in high-dimensional integer lattices; these protocols offer strong security proofs, relatively small key sizes (on the order of tens of kilobytes), and efficient software profiles. Code-based algorithms—such as Classic McEliece—derive security from decoding random linear error-correcting codes, boasting exceptionally fast encryption and decryption at the expense of larger public keys (multiple megabytes). Hash-based signatures, exemplified by SPHINCS+, use hierarchical constructions over collision-resistant hash functions to provide stateless, forward-secure authentication without relying on number-theoretic assumptions. Other contenders include multivariate-quadratic schemes and isogeny-based protocols, each with unique trade-offs in performance, key size, and implementation complexity.
Strategic Importance
For national governments, migrating to PQC is not merely a software update—it requires end-to-end integration across hardware modules, network protocols, and device firmware. Embedding lattice-based key-exchange into VPN appliances and TLS libraries ensures that diplomatic traffic and server-to-server APIs remain confidential even if adversaries archive intercepted ciphertexts for future quantum decryption. Code-based algorithms are well suited for securing satellite links and constrained Internet-of-Things (IoT) devices, where decryption speed and resistance to side-channel leakage are paramount. Hash-based signatures provide a quantum-secure method for firmware authentication on critical sensors and control systems. By coordinating national standards bodies with defense and civilian agencies, countries can orchestrate smooth transitions that safeguard both classified networks and public digital services.
Leading Nations in PQC Development
United States drives PQC standardization through NIST’s multi-year competition, championing lattice and hash-based candidates, while defense agencies fund hardware acceleration research at National Labs to integrate PQC into cryptographic modules. China pursues indigenous PQC schemes—such as a ring-learning-with-errors variant—and is rolling out PQC-capable VPNs within its government networks. Germany and the broader European Union coordinate under ETSI and the EU’s Cybersecurity Industrial, Technology and Research Competence Centre to harmonize PQC standards across member states, with pilot deployments on 5G core networks. Japan leads in silicon design for PQC accelerators, embedding lattice-based primitives into smart-card chips for banking and identification. India’s Centre for Development of Advanced Computing (C-DAC) is prototyping PQC libraries for secure e-governance platforms and national identity systems.
Technical and Policy Challenges
Implementing PQC at scale involves addressing large key and signature sizes, ensuring side-channel resistance in embedded devices, and updating legacy systems without service interruptions. Cross-border interoperability demands alignment on chosen algorithms and parameter sets, as divergent national standards could fragment global communications. Export-control frameworks must evolve to accommodate PQC software and hardware, balancing security with industrial competitiveness.
Future Outlook
As research advances toward hybrid schemes—combining classical and post-quantum primitives for graceful security transitions—nations will increasingly adopt agile cryptographic architectures capable of algorithm agility and rapid updates. Hardware support for lattice-based operations will mature, driving key-exchange latencies below classical benchmarks. International collaboration on PQC certification labs and shared testbeds will accelerate trustworthy deployments. By investing in national PQC research, workforce development, and cross-sector integration, governments will fortify their digital ecosystems against the quantum threat and preserve the confidentiality and integrity of critical communications well into the quantum era.